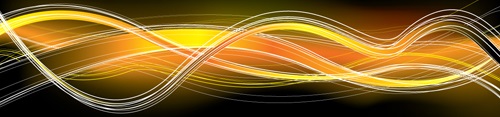
Quantum Metamaterials
The main aim of QUANTUMMETALINK project, a research project supported by the European Reserch Council via
an ERC Consolidator grant, is to develop an integrated theoretical and
computational research program in an emerging area of metamaterials
research, namely Quantum Metamaterials. The fundamental idea in this
area of research is that remarkable physical properties beyond those
available in naturally occurring materials can be achieved by designing
the meta-constituents of the metamaterial and structuring it at a scale
comparable or smaller than the optical wavelength. In this context, a
new paradigm in metamaterials research emerges when the building blocks
of metamaterials are quantum resonators, e.g., quantum dots, quantum
dot molecules, graphene disks coupled to quantum dots, quantum
nanowires, and quantum rings, case in which the macroscopic properties
of quantum metamaterials are determined by the quantum properties of
their basic constituents. We have organised our research in this field
along three broad, synergistically integrated themes: the development
of a general theory of the effective, macroscopic properties of quantum
metamaterials, the development of a set of numerical methods and
software tools for ab initio
simulation of fundamental physical properties of quantum metamaterials,
and the exploration of new science and potentially novel technological
applications of quantum metamaterials.
|
Publications
|
 |
Giant Enhancement of Nonlinear Optical Interactions in 2D Materials |
Remarkable
optical and electrical properties of two-dimensional (2D) materials,
such as graphene and transition-metal dichalcogenide (TMDC) monolayers,
offer vast technological potential for novel and improved
optoelectronic nanodevices, many of which rely on nonlinear optical
effects in these 2D materials. Recently, we introduced a highly
effective numerical method for efficient and accurate description of
linear and nonlinear optical effects in nanostructured 2D materials
embedded in periodic photonic structures containing regular
three-dimensional (3D) optical materials, such as diffraction gratings
and periodic metamaterials - see below a schematic of such an optical
device. Our method builds upon the rigorous coupled-wave analysis and
incorporates the nonlinear optical response of 2D materials by means of
modified electromagnetic boundary conditions. This allows one to reduce
the mathematical framework of the numerical method to an inhomogeneous
scattering matrix formalism, which makes it more accurate and efficient
than previously used approaches. To illustrate the versatility of our
numerical method, we used it to investigate the linear and nonlinear
multiresonant optical response of 2D-3D heteromaterials for enhanced
and tunable second- and third-harmonic generation. In particular, by
employing a TMDC monolayer optically coupled to a patterned slab
waveguide, we demonstrate orders-of-magnitude enhancement of the
intensity of generated second-harmonic.
|
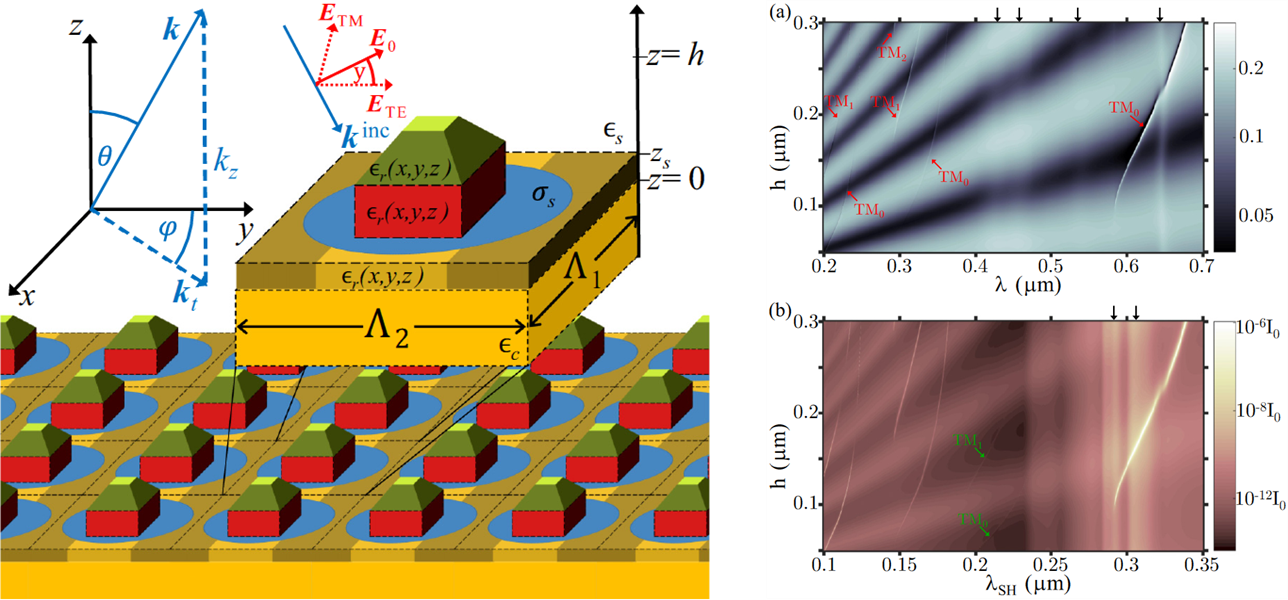
|
|
|
|
|
Nonlinear Optical Diffraction Gratings Based on 2D Materials |
Intriguing
and unusual physical properties of graphene offer remarkable potential
for advanced, photonics-related technological applications,
particularly in the area of nonlinear optics at the deep-subwavelength
scale. In this context, we have recently used an in-house developed
numerical method to illustrate an efficient mechanism that can lead to
orders-of-magnitude enhancement of the third-harmonic generation in
graphene diffraction gratings. In particular, we demonstrate that by
taking advantage of the geometry dependence of the resonance wavelength
of localized surface-plasmon polaritons of graphene ribbons and discs
one can engineer the spectral response of graphene gratings so that
strong plasmonic resonances exist at both the fundamental frequency and
third-harmonic. As a result of this double-resonant mechanism for
optical near-field enhancement, the intensity of the TH can be
increased by more than six orders of magnitude.
|
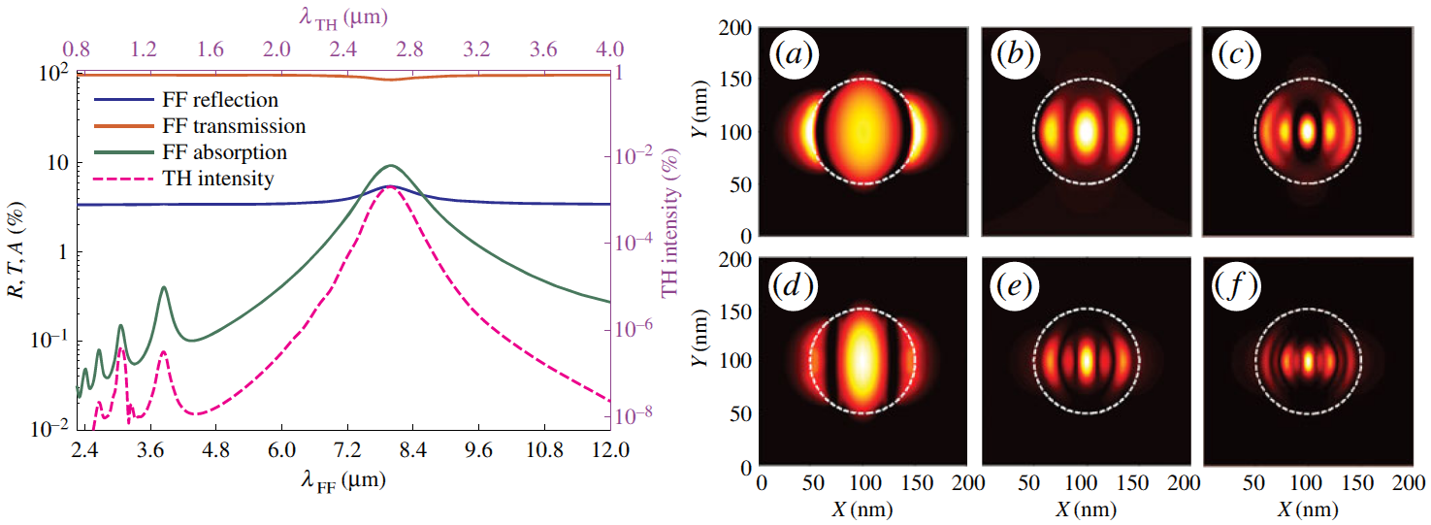
|
|
|
|
|
Quantum Plasmons in Single and Interacting Graphene Nanoflakes
|
In
order to develop a computational framework to investigate and predict
the optical properties of 2D quantum metamaterials, we started with the
well-known single layer material – graphene and nanostructures made out
of it. This choice has been motivated by the technical feasibility of
synthetizing molecular-sized graphene nanoflakes, which could be the
constituting bulding blocks of 2D quantum metamaterials. At the
molecular scale the light-matter interaction is controlled by quantum
mechanics, therefore it is expected that the quantum mechanical effects
in graphene nanoflakes significantly influence the optical response of
metamaterials based on such nanostructures. Hence the special name,
quantum metamaterial can be given to this class of metamaterials. At
the same time, it is also possible to produce large (but subwavelength
size) graphene nanostructures using cutting techniques. If these
nano-structures are used as metamaterial components the theoretical
modelling can be performed based on classical electrodynamics.
Therefore by using graphene one would have the practical means to
investigate the transition between different physical regimes. To this
end, we have studied the optical spectra for a variety of single and
coupled graphene nanoflakes using time-dependent density functional
theory within generalized gradient approximation as well as the
dependence of these spectra on the shape and size of the graphene
nanoflakes. Among other things, our analysis has shown that the
physical properties of quantum plasmons of graphene nanoflakes are
markedly distinct from those of their classical counterparts, e.g.,
their energy obeys a different power-law dependence on the nanoflake
size. |
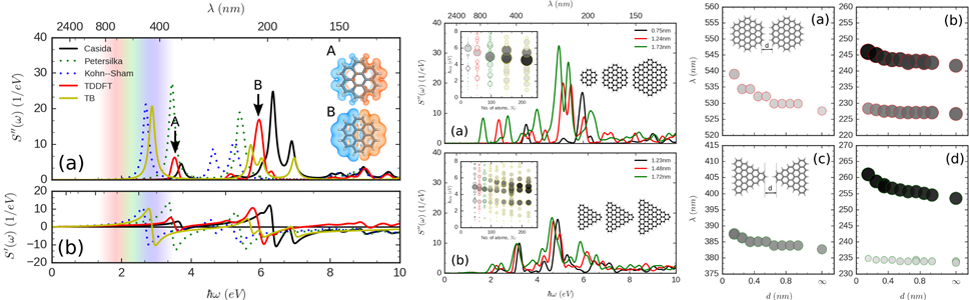
|
|
|
|
|
Molecular Sensors based on Quantum Graphene Plasmons
|
The
graphene nanoflakes discussed above and dimers formed from such
graphene nanoflakes, can be viewed as very large molecular systems,
characterized by specific optical absorption spectra. As a result, when
a specific molecule is placed on top of a graphene nanoflake or
in-between two graphene nanoflakes, as it is schematically illustrated
in the figure below, one expects that the optical absorption spectrum
of the combined physical system will be modified in a certain way,
which is specific to the particular molecule added to the system and
the shape and configuration of the graphene nanoflake and dimers made
of graphene nanoflakes. Therefore, these changes of the optical spectra
can be viewed as signatures of the specific molecules interacting with
graphene nanoflakes and thus can be used to detect the molecules. A
first step towards understanding how these quantum plasmon sensors
work is to figure out the extent to which a molecular bridge linking
two graphene nanoflakes affects the plasmon tunneling between the two
graphene dots. In performing this analysis, as illustrated below, the
optical spectra of the corresponding nanostructures prove to be
powerful tools.
|
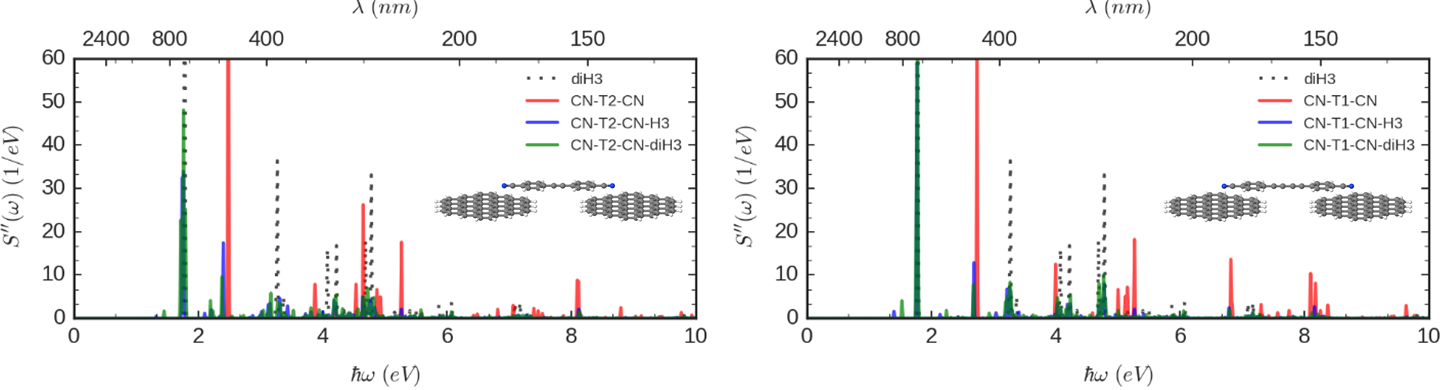
|
|
|
|