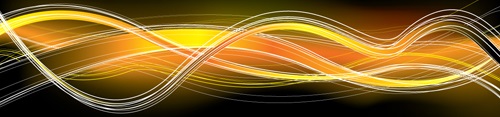
|
 |
Theoretical Modeling of Optical Pulse Dynamics in Silicon Photonic Nanowires
|
The
distinctive features of silicon photonic nanowires are that they have
extremely large third-order susceptibility and strongly enhanced
dispersive properties. As a result, the relatively low threshold powers
for nonlinear optical effects in these wires make them potential
candidates for functional on-chip nonlinear optical devices of just a
few millimeters in length; conversely, the absence of nonlinear optical
impairment is important for the use of silicon wires in on-chip
interconnects. In addition, the characteristic length scales of linear
and nonlinear optical effects in Si wires are markedly different from
those in commonly used optical guiding systems, such as optical fibers
or photonic crystal fibers, and therefore guiding structures based on
silicon wires represent ideal optical media for investigating new and
intriguing physical phenomena. In this context, we are actively
involved in several projects aimed to developed rigorous, comprehensive
theoretical models and software tools that describe the propagation of
optical pulses in silicon-based deep sub-wavelength waveguides. In
particular, we seek to develop models that account for linear optical
effects, such as material and waveguide dispersion, free-carrier
absorption, and free-carrier induced dispersion, nonlinear effects, such
as self- and cross-phase modulation, two-photon absorption, spontaneous
and stimulated Raman scattering, and the frequency dispersion of the
effective optical nonlinearity of the waveguide, as well as the mutual
interaction between the optical field and the free-carriers. This work
is an ongoing collaboration with Prof. Richard Osgood's group from Columbia University's Department of Electrical Engineering.
|
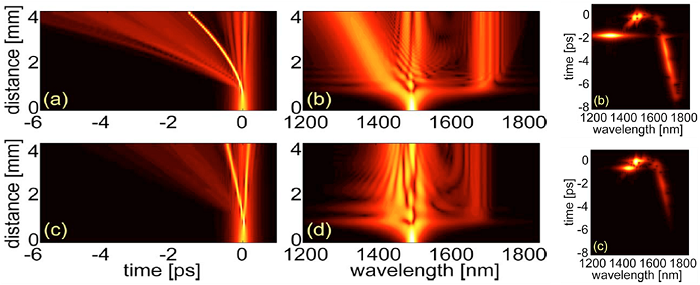
|
Relevant publications:
- X. Chen, N. C. Panoiu, and R. M. Osgood, Jr., Theory of Raman-mediated pulsed amplification in silicon-wire waveguides, IEEE J. Quantum Electron. 42, 160 (2006). [pdf]
- J. I. Dadap, N. C. Panoiu, X. Chen, I-W.
Hsieh, X. Liu, C.-Y. Chou, E. Dulkeith, S. J. McNab, F. Xia, W. M. J.
Green, L. Sekaric, Y. A. Vlasov, and R. M. Osgood, Jr., Nonlinear-optical phase modification in dispersion-engineered Si photonic wires, Opt. Express 16, 1280 (2008). [pdf]
- N. C. Panoiu, X. Liu, and R. M. Osgood, Jr., Self-steepening of ultrashort pulses in silicon photonic nanowires, Opt. Lett. 34, 947 (2009) (also featured in the Virtual Journal of Nanoscale Science and Technology 19 (17), April 27, 2009 and the Virtual Journal of Ultrafast Science 8 (5), May, 2009). [pdf]
- R. M. Osgood, Jr., N. C. Panoiu, J. I. Dadap, X. Liu, X. Chen, I-W. Hsieh, E. Dulkeith, W. M. J. Green, and Y. A. Vlasov, Engineering
nonlinearities in nanoscale optical systems: physics and applications
in dispersion-engineered silicon nanophotonic wires, Adv. Opt. Photon. 1, 162 (2009). [pdf]
- J. B. Driscoll, R. R. Grote, X. Liu, J. I. Dadap, N. C. Panoiu, and R. M. Osgood, Jr., Directionally anisotropic Si nanowires: on-chip nonlinear grating devices in uniform waveguides, Opt. Lett. 36, 1416 (2011). [pdf]
|
|
|
|
Generation of Optical Similaritons and Optical Pulse Compression in Silicon Photonic Nanowires |
Due
to their strongly enhanced frequency dispersion and optical
nonlinearity, silicon photonic nanowires represent an ideal test bed
for exploring new physical regimes of optical pulse dynamics in
nonlinear media. In particular, these photonic nanodevices provide an
effective chip-scale aproach for optical pulse generation as well as
temporal and spectral reshaping of optical pulses. In this context, in
collaboration with Prof. Richard Osgood's group from Columbia University's Department of Electrical Engineering,
we have recently demonstrated that by tapering photonic nanowire
waveguides made of silicon one can generate parabolic self-similar
optical pulses both at telecom and mid-IR wavelengths. Our
computational study is based on a rigorous theoretical model, which
fully describes the influence of linear and nonlinear optical effects
on pulse propagation in silicon photonic wire waveguides with
arbitrarily varying transverse size. Numerical simulations demonstrate that in
the normal dispersion regime Gaussian pulses evolve naturally into
parabolic pulses, upon their propagation in millimeter-long tapered
silicon photonic wire waveguides, the efficiency of this pulse
reshaping process being strongly dependent on the spectral and pulse
parameter regime in which the device operates. In addition, we have
demonstrated that one could achieve temporal compression of ultra-short
optical pulses by more than three times in millimetre-long
adiabatically tapered silicon photonic nanowire waveguides when the
optical pulses propagate in the soliton regime.
|
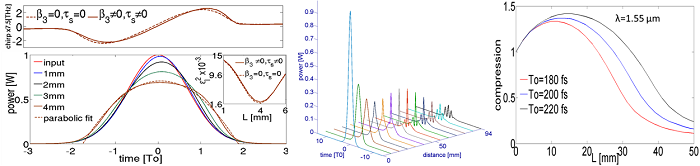
|
Relevant publications:
- S. Lavdas, J. B. Driscoll, H. Jiang, R. R. Grote, R. M. Osgood, Jr, and N. C. Panoiu, Generation
of parabolic similaritons in tapered silicon photonic wires: comparison
of pulse dynamics at telecom and mid-infrared wavelengths, Opt. Lett. 38, 3953 (2013). [pdf]
- S. Lavdas, J. B. Driscoll, R. R. Grote, R. M. Osgood, Jr, and N. C. Panoiu, Analysis of optical pulse compression in tapered silicon photonic wires, Optics Express 22, 6296 (2014). [pdf]
|
|
|
|
Optical Pulse Propagation in Photonic Crystal Slab Waveguides
|
An
effective approach to enhance both the frequency dispersion and the
optical nonlinearity of subwavelength silicon based waveguiding
nanodevices is to pattern them at subwavelength scale. This provides an
efficient design tool for engineering the optical properties of
photonic waveguiding nanodevices and, equally important, it allows one
to design nonlinear photonic structures in which optical pulses
propagate in the slow-light regime. Guided by these ideas, recently we
have developed a comprehensive theoretical description of the
propagation of optical pulses in one-dimensional waveguides consisting
of a line defect in a photonic crystal slab waveguide made of silicon.
We have incorporated in our analysis linear optical effects, such as
group-velocity dispersion and optical losses, as well as nonlinear
effects induced by the Kerr nonlinearity of the photonic crystal. We
have also included in our model the free-carrier dispersion and
free-carrier induced optical losses, and thus studied the influence of
free-carriers generated through two-photon absorption on the optical
pulse dynamics. Our analysis has revealed that important quantities,
such as the pulse group-velocity, waveguide dispersion coefficients, or
the waveguide nonlinear coefficient are strongly affected by the
periodic nature of the guiding structure. Equally important, we have
demonstrated that both linear and nonlinear effects are stronger in the
case of slow-light modes, with the nonlinear effects being enhanced
more as compared to the linear ones. This work represents an ongoing
collaboration with Prof. Chee Wei Wong's group from the Fu Foundation School of Engineering and Applied Science at Columbia University. |
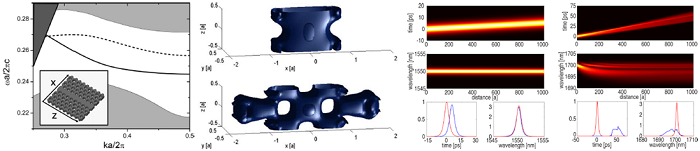
|
Relevant publications:
- N. C. Panoiu, J. F. McMillan, and C. W. Wong, Theoretical Analysis of Pulse Dynamics in Silicon Photonic Crystal Wire Waveguides, IEEE J. Sel. Top. Quantum Electron. 16, 257 (2010). [pdf]
- J. B. Driscoll, N. Ophir, R. R. Grote, J. I. Dadap, N. C. Panoiu, K. Bergman, and R. M. Osgood, Jr, Width-modulation of Si photonic wires for quasi-phase-matching of four-wave-mixing: experimental and theoretical demonstration, Opt. Express 20, 9227 (2012). [pdf]
|
|
|
|
Photonic Metamaterials with Zero Index of Refraction
|
One
of the most remarkable properties of metamaterials is that, unlike
naturally occurring materials, their physical properties can be easily
engineered or tuned by simply modifying the structure and/or materials
properties of their building blocks. One such example of a metamaterial
with unusual electromagnetic properties is an optical medium whose
index of refraction is equal to zero. These metamaterials have unique
optical properties, including a new type of surface states and gap
solitons, unusual transmission and emission properties, complete
photonic bandgaps, a phase-invariant propagating electromagnetic field
(a temporally "frozen" electromagnetic field that is spatially
inhomogeneous), and an omnidirectional bandgap that is insensitive to
wave polarization, incidence angle, structure periodicity, and
structural disorder. Recently, we have demonstrated theoretically that
under certain circumstances a photonic super-lattice consisting of
alternating layers of homogeneous media with positive index of
refraction and photonic crystal layers with negative index of
refraction emulates the properties of an optical medium with zero index
of refraction. In a collaborative project with Prof. Chee Wei Wong's group from the Fu Foundation School of Engineering and Applied Science at Columbia University, these ideas have
recently been experimentally validated. In adition,
chip-scale photonic devices based on these zero-n metamaterials,
including optical filters and interferometers, have been
demonstrated.
|
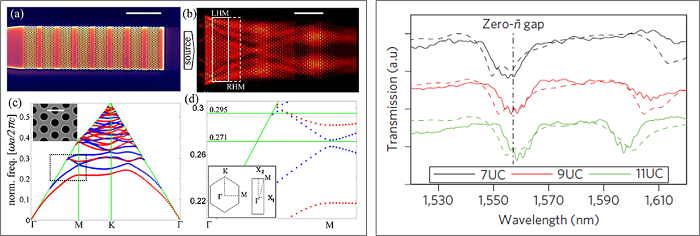
|
Relevant publications:
- N. C. Panoiu, R. M. Osgood, Jr., S. Zhang, and S. R. J. Brueck, Zero–n band-gap in photonic crystal superlattices, J. Opt. Soc. Am. B 23, 506 (2006). [pdf]
- S. Kocaman, R. Chatterjee, N. C. Panoiu, M. B. Yu, R. M. Osgood, Jr., D. L. Kwong, and C. W. Wong, Observations of Zero-order Bandgaps in Negative Refraction Photonic Crystal Superlattices at the Near-Infrared, Phys. Rev. Lett. 102, 203905 (2009) (also featured in the Virtual Journal of Nanoscale Science and Technology 19 (23), June 8, 2009). [pdf]
- S. Kocaman, M. S. Aras, P. C. Hsieh, J. F.
McMillan, C. G. Biris, N. C. Panoiu, M. B. Yu, D. L. Kwong, A. Stein,
and C. W. Wong, Zero phase delay in negative-index photonic crystal superlattices, Nature Photonics 5, 499 (2011). [pdf]
- S. Kocaman, M. S. Aras, N. C. Panoiu, Ming Lu, and C. W. Wong, On-chip optical filters with designable characteristics based on an interferometer with embedded silicon photonic structures, Opt. Lett. 37, 665 (2012). [pdf]
- C. W. Wong, S. Kocaman, M. S. Aras, P.
C. Hsieh, J. F. McMillan, C. G. Biris, N. C. Panoiu, M. B. Yu, D. L.
Kwong, and A. Stein, Negative Index Photonic Crystals Superlattices and Zero Phase Delay Lines, in Photonic Crystals – Innovative Systems, Lasers and Waveguides, Ed. A. Massaro (InTech, 2012). [pdf]
|
|
|